Agriculture
Plant Biotechnology
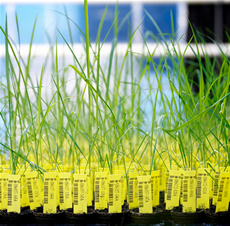 |
Plant Biotechnology |
Plant biotechnology may be defined as the application of knowledge obtained from study of the life sciences to create technological improvements in plant species. By this very broad definition, plant biotechnology has been conducted for more than ten thousand years.
The roots of plant biotechnology can be traced back to the time when humans started collecting seeds from their favorite wild plants and began cultivating them in tended fields. It appears that when the plants were harvested, the seeds of the most desirable plants were retained and replanted the next growing season.
While these primitive agriculturists did not have extensive knowledge of the life sciences, they evidently did understand the basic principles of collecting and replanting the seeds of any naturally occurring variant plants with improved qualities, such as those with the largest fruits or the highest yield, in a process that we call artificial selection. This domestication and controlled improvement of plant species was the beginning of plant biotechnology.
This very simple process of selectively breeding naturally occurring variants with observably improved qualities served as the basis of agriculture for thousands of years and resulted in thousands of domesticated plant cultivars that no longer resembled the wild plants from which they descended.
The second era of plant biotechnology began in the late 1800?s as the base of knowledge derived from the study of the life sciences increased dramatically.
In the 1860?s Gregor Mendel, using data obtained from controlled pea breeding experiments, deduced some basic principles of genetics and presented these in a short monograph modestly titled ?Versuche über Pflanzenhybriden? (in Verhandlungen des naturforschenden Vereins, 1866; Experiments with Plant-Hybridisation, 1910).
 | Plant Biotechnology: The Genetic Manipulation of Plants |
 |  | Plant Biotechnology and Agriculture: Prospects for the 21st Century |
 |
In this publication, Mendel proposed that heritable genetic factors segregate during sexual reproduction of plants and that factors for different traits assort independently of each other.
Mendel?s work suggested a mechanism of heritable factors that could be manipulated by controlled breeding of plants through selective fertilization and also suggested that the pattern of inheritance for these factors could be analyzed or, in some cases, predicted by the use of mathematical statistics.
These findings complemented the work of Charles Darwin, who expounded the principles of descent with modification and selection as the chief factor of evolutionary change in his 1859 book On the Origin of Species by Means of Natural Selection.
The application of these principles to agriculture resulted in deliberately produced hybrid varieties for a large number of cultivated plants via selective fertilization. These artificially selected hybrids soon began to benefit humankind with tremendous increases in both the productivity and the quality of food crops.
Genetic Engineering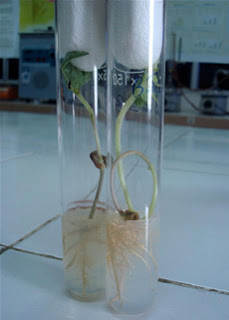 |
Genetic Engineering |
The third era of plant biotechnology involves a drastic change in the way crop improvement may be accomplished, by direct manipulation of genetic elements (genes).
This process is known as genetic engineering and results in plants that are called genetically modified organisms (GMOs), to distinguish them from plants that are produced by conventional plant-breeding methods.
Genetically modified plants can contribute desirable genes from outside traditional breeding boundaries. Even genes from outside the plant kingdom can now be brought into plants. For example, animal genes, including human genes, have been transferred into plants, a feat not replicated in nature.
Public ConcernIt is perhaps this lack of natural boundaries for genetic exchange that seems so foreign to conventional scientific thought and that makes plant genetic engineering controversial.
The thought of taking genes from animals, bacteria, viruses, or any other organismand putting theminto plants, especially plants consumed for food, has raised a host of questions among concerned scientists and public alike.
Negative public perception of genetically modified crops has affected the development and commercialization of many plant biotechnology products, especially food plants. While there are dozens of genetically engineered plants ready for field production, public pressure has delayed the release of some of these plants and has caused the withdrawal of others from the marketplace.
This public concern also appears to be driving increased government review of products and decreased government funding for plant biotechnology projects in Europe. Negative public perceptions do not seem to be as strong in Asia, since the pressures of feeding large populations tend to outweigh the perceived risks.
The social climate of the United States toward biotechnology, although guarded, appears to be less apprehensive than that of most European countries. Therefore, many agricultural biotechnology projects have moved from European countries to U.S. laboratories.
Economic GoalsTo what end are humans genetically engineering plants? This is an essential question for researchers, executives of biotechnology companies, and consumers at large.
Before addressing technical questions about how to apply biotechnology, the desired goals must be clearly defined. The general goals of plant biotechnology appear to be
- economic improvement of existing products,
- improvement of human nutrition, and
- development of novel products from plants.
Economic improvements include increases in yield, quality, pest resistance, nutritional value, harvestability, or any other change that adds value to an established agricultural product.
Examples of this category include insect-protected tomatoes, potatoes, cotton, and corn; herbicide-resistant canola, corn, cotton, flax, and soybeans; canola and soybeans with genetically altered oil compositions; virus-resistant squash and papayas; and improved ripening tomatoes. All these examples were introduced to agriculture in the later half of the 1990?s.
Nutritional GoalsAdditionally, some products appearing in the scientific literature but awaiting commercialization have the potential to dramatically improve human nutritional deficiencies, which are especially prevalent in developing countries.
These products include ?golden rice,? genetically modified rice that produces carotenoids, a dietary source of vitamin A. Golden rice has the potential to prevent vitamin A deficiency in developing countries, where this vitamin deficiency is a leading cause of blindness.
Researchers are also using genetic engineering to increase the amount of the iron-storing protein ferritin in seed crops such as legumes. Iron deficiency, which affects 30 percent of the human population, can impair cognitive development and cause other other health problems. This proposed enhancement of iron content in consumable plant products could help more than a billion people who suffer from chronic iron deficiency.
Novel ProductsNovel products include those not traditionally associated with plants and are limited only by imagination and currently available techniques. These include the production of plastics, vaccines, antibodies, human blood proteins, and new pharmaceuticals.
One project has involved the production of hepatitis B vaccine in transgenic tomatoes. This project, which underwent clinical trials in the late 1990?s, has the potential to provide a simple and inexpensive means of vaccinating people against hepatitis B.
By oral administration of tomato juice containing the vaccine protein, humans are thought to develop an immune response that may protect them from infection by the hepatitis B virus. Hepatitis B is epidemic in Asia and increasing at an alarming rate in the rest of the world. The disease ultimately causes liver disease, cancer, and death in millions of infected people.
Plant Tissue CulturesCentral to plant biotechnology is the use of in vitro methods. Researchers use plant tissue cultures, for example, to grow plant cells on sterile nutrient media. Countless recipes for these nutrient media exist. The choice ofwhich one to use is based on the plant species and the tissue type to be grown.
All such media contain at least some of the important nutitional elements, such as nitrogen, potassium, calcium, magnesium, sulfur, phosphorus, iron, boron, manganese, zinc, iodine, molybdenum, copper, and cobalt, usually in the form of inorganic salts or as metal chelates, and an organic energy source, such as sucrose. The media may also contain vitamins, hormones, and other ingredients, depending on the intended use.
To initiate plant tissue culture, a piece of a living plant is excised and disinfected using a chemical disinfectant.
This piece of plant tissue, called an explant, is placed on a sterile plant tissue culture medium to grow. Many plant tissues may be used to obtain explants for plant tissue culture, including those from leaves, petioles, shoots, tubers, roots, and meristematic regions.
When an explant is placed in the sterile tissue culture medium, cells that are not terminally differentiated will grow and divide. If plant hormones are included in the recipe, the plant cells can be coaxed to develop into different types of tissues or organs.
By using a succession of media containing different hormones, it is possible to regenerate whole plants from single cells. The choice of tissue used for the explant and the choice of hormones included in the tissue culture medium depend on the desired result.
MicropropagationMicropropagation, another biotechnology technique, is the production of many clonal plants using tissue culture methods. By means of micropropagation, it is possible to generate many thousands of plant clones using tissue explants obtained froma single parent plant. The main advantage to micropropagation is the potential of producing thousands of exact copies of a plant with desirable traits.
Micropropagation is especially important for rare plants, genetically engineered plants, and plants that have sexual reproductive problems. Many plant species are now routinely propagated by micro propagation methods, including orchids, ferns, many flowering ornamentals, and vegetable plants.
Steps in Genetic EngineeringThe first genetically engineered plants, tobacco plants, were reported in the scientific literature in 1984. Since 1984 there have been thousands of genetically engineered plants produced in laboratories worldwide. The process of genetically engineering a plant involves several key steps:
- isolating the genetic sequence (gene) to be placed from its biological source
- placing the gene in an appropriate vehicle to facilitate insertion into plant cells
- inserting the gene into the plant in a process known as plant transformation
- selecting the few plant cells that contain the new gene (transformed cells) out of all the plant cells in the explant
- multiplying the transformed cells in sterile tissue culture
- regenerating the transformed cells into a whole plant that can grow outside the tissue culture vessel
The gene or genes to be placed in the plant may be obtained from virtually any biological source: animals, bacteria, fungi, viruses, or other plants. Placing genes into an appropriate vehicle for transfer into a plant involves using various molecular biology techniques, such as restriction enzymes and ligation, to essentially ?cut and paste? the gene or genes of interest into another DNA molecule, which serves as the transfer vehicle (vector).
Plant Transformation MethodsCurrently plant transformation with foreign genes may be accomplished by several proven methods, including bacteria-mediated transfer,microparticle bombardment, electroporation, microinjection, sonication, and chemical treatment.
By far, the most often utilized method of plant transformation involves the use of naturally occuring plant pathogenic bacteria from the genus Agrobacterium. In nature, this bacterium infects plants and transfers some of its own bacterial DNA into the plant.
Through the action of proteins produced by the bacteria, bacterial DNA is made to integrate permanently into the plant?s own genomic DNA. Expression of the bacterial DNA in the plant causes the plant to produce unusual quantities of plant hormones and other compounds, called opines, which provide food for the bacteria.
The unusual quantities of plant hormones around the infection site cause the plant cells to grow abnormally, producing characteristic tumors. Scientists have harnessed this pathogenic bacterium to insert genes into plants by deleting the bacterial genes that cause tumors in the plant and then inserting desirable genes in their place.
When the modified Agrobacterium infects a plant, it transfers the desirable genes into the plant genome instead of causing tumors. The desirable genes become a permanent part of the plant genome, and expression of these genes in plant cells produces desirable products.
One major drawback of the Agrobacterium method is that insertion of bacterial DNA into the plant genome is essentially random. The gene may not be efficiently transcribed at its location, or the insertion of bacterial DNA may knock out an important plant gene by inserting in the middle of it or both may occur. Therefore, the fact that a cell is genetically transformed does not guarantee that it will perform as desired.
Microparticle bombardment is the introduction of foreign DNA constructs into plant cells by attaching the DNA to small metal particles and blasting the particles into plant cells using either a compressed air gun or a gun powered by a 0.22 caliber gun cartridge.
This is truly a ?brute force? method of introducing DNA into a cell that inadvertently causes many lethal casualties among the bombarded plant cells. However, some plant cells blasted with the DNA-containing metal particles will recover and survive.
The plant cells may express the DNA for only a short time (transient expression), because the DNA does not readily integrate into the plant genome, but occasionally the foreign DNA may spontaneously recombine into the plant genome and become permanent.
Other ways of introducing foreign DNA into plant cells include electroporation, microinjection, sonication, and chemical treatment. These methods are not used extensively, because they generally require the production of protoplasts (plant cells that lack their cell walls) from plant cells before transformation.
To create protoplasts, the plant cell wall is removed by digestion with the enzymes cellulase and pectinase. Protoplasts are fragile structures, but the absence of a cell wall is desirable because it leaves only the plasma membrane as a barrier to foreign DNA entering a plant cell.
Electroporation uses very brief pulses of high-voltage electrical energy to create temporary holes in the plasma membrane through which the foreign DNA can pass. Micro injection involves physically injecting a small amount of DNA into a plant cell using a microscope and an extremely fine needle.
Sonication uses ultrasonic waves to punch temporary holes in the plasma membrane; this method is therefore similar to electroporation. Chemical treatment involves the use of polyethylene glycol to render the plasma membrane permeable to foreign DNA.
All the transformation procedures produce only a few transformed cells out of the millions of cells in an explant, so selection of transformed cells is essential.
Selection of Transformed Plant CellsSelecting the few transformed plant cells out of all the plant cells in an explant requires some advance planning. Most foreign DNA constructs introduced into a plant are designed and built to contain additional genes that function as selectable markers or reporter genes. Selectable markers include genes for resistance to antibiotics or herbicides.
Plant cells containing and expressing these genes will be tolerant of antibiotics or herbicides added to the plant tissue culture media, while the nontransformed plant cells will be killed off. The surviving cells in the tissue culture media are mostly transformed.
Instead of selectable markers, reporter genes may be used. Reporter genes induce an easily observable trait to transformed plant cells that facilitates the physical isolation of these cells.
Reporter genes include beta-glucuronidase, luciferase, and plant pigment genes. Beta-glucuronidase (commonly known as GUS) allows the plant cells expressing this gene to metabolize colorigenic substrates while nontransformed plant cells cannot.
To use this test, researchers treat a small amount of plant tissue with the colorigenic chemical substrate. If the cell turns color (blue) it is known to be transformed and expressing the GUS gene. If the cell does not turn color, it probably is not transformed.
Another reporter gene is luciferase, an enzyme isolated from fireflies. Luciferase makes plant cells glow in the presence of certain chemicals if the gene is present; hence, transformed cells glow, where as non transformed cells do not glow.
Plant pigment genes, such as anthocyanin pigment genes, occur naturally in plants and produce pigments that impart color to flowers. Inclusion of these pigment genes as reporter genes will allow transformed plant cells to be selected by their color.
Transformed cells have color, while non transformed cells remain colorless. Both selectable markers and reporter genes allow selection of cells into which genes have been successfully inserted and are operating properly.
Regenerating Whole Transformed Plants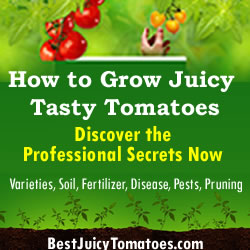 |
How to grow juicy tasty tomatoes |
After successfully getting a gene construct into a plant cell and selecting the transformed cells, it is possible to get the plant cells to multiply in tissue culture. Also, by treating the plant cells with combinations of plant hormones, the cells are made to differentiate into various plant organs or whole plants.
For example, treating transformed plant cells with a high concentration of the plant hormone cytokinin causes shoots to develop. Transferring these shoots to anothermedium, one that is high in the plant hormone auxin, will cause roots to develop on the shoots.
In this way a whole transgenic plant may be regenerated from transformed plant cells. Once a transformed plant is regenerated in tissue culture, the plant may be transferred to a climate-controlled greenhouse, where it can grow to maturity.
Future generations of transgenic plants may then be propagated sexually via seeds or asexually via vegetative propagation methods. Often transgenic plants must be grown in containment greenhouses to prevent accidental release into the environment.
In such high-tech greenhouses, all factors contributing to optimal plant growth?lighting, temperature, humidity, nutrients, and other environmental conditions?are tightly controlled. Often hydroponic systems, which use a solution of plant nutrients as a growth medium in place of soil, are employed to control all aspects of plant nutrition.
-
The Benefits Of Agricultural
Agricultural biotechnology is any technique in Which living organisms, or parts of organisms are altered to make or modify agricultural products, to improve crops, or develop microbes for specific uses in agricultural processes. Simply put, when the...
-
Bacterial Genetics
Bacterial GeneticsBacterial genetics is the study of the genetic material of bacterial DNA, which can provide valuable insights into the process of mutation because of bacteria?s rapid rate of reproduction. Plants were the original candidates for genetic...
-
Cloning Of Plants
Plant cloning is the production of a cell, cell component, or plant that is genetically identical to the unit or individual from which it was derived. The term?clone? is derived from the Greek word klon, meaning a slip or twig. Hence, it is an appropriate...
-
Genetically Modified Bacteria
Genetically Modified BacteriaBacteria may be genetically modified through the introduction of recombinant DNA molecules into their cells. Such bacteria may be used to produce human insulin or introduce disease-resistant genes into plants, as well as numerous...
-
Why Gmos Are Dangerous?
Genetically modified organisms (GMO?s) are a broad group of plants, animals, and bacteria that are engineered for a wide variety of applications ranging from agricultural production to scientific research. The types of potential hazards posed by GMO?s...
Agriculture