Agriculture
Evolution of Cells
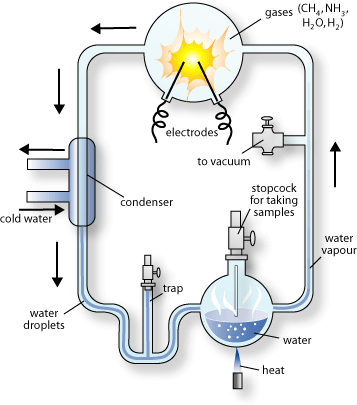 |
Evolution of Cells |
The earliest cells evolved sometime early in the Precambrian era,which includes the first four billion years of Earth?s history. Attempts to understand life?s origins are difficult, as there are very few clues left in the fossil record from those early times.
The hypotheses and models of the origin of life that have been developed are based on contemporary understanding of how life works at the molecular and cellular levels and on assumptions about the conditions on Earth three billion to four billion years ago.
One assumption made about the origins of life involves the composition of the atmosphere shortly after the earth was formed. According to this assumption, the earth?s atmosphere at this time contained very little free oxygen. It was an atmosphere perhaps made primarily of methane, ammonia, carbon dioxide, nitrogen, carbon monoxide, and water vapor.
The first organisms are believed to have been anaerobic and did not require oxygen for respiration. This early atmosphere lacked a protective shield of ozone, which is derived from oxygen and which absorbs ultraviolet radiation from the sun.
Intense ultraviolet radiation is lethal, and early forms of life may have evolved in water deep enough to avoid it. In some later stages of chemical evolution, however, ultraviolet radiation may actually have driven molecular interactions, producing more complex structures that were forerunners to living organisms.
Building Blocks of LifeThe basic elements found in organic compounds are carbon, oxygen, hydrogen, nitrogen, phosphorus, and sulfur. These elements are also the main components of living cells and are the most plentiful elements in the solar system. The basic materials for the development of life were present on the early earth.
Scientists have discovered that some of these building blocks, especially molecules of hydrogen sulfide, hydrogen cyanide, methanol, acetic acid,methyl formate, and a simple sugar called glycol aldehyde, exist in space and may have been brought to Earth by comets passing close by the plant.
These compounds were, in fact, given off as Comet Hale-Bopp passed through the earth?s solar system during 1995-1997. Perhaps comets ?seeded? the earth with inorganic and organic compounds that triggered the chemical evolution that led to life itself.
Proteins and Amino AcidsOne of the components of life is proteins. Proteins are made of even more basic organic molecules called amino acids. Proteins act as building materials for an organism?s body and function in chemical reactions within an organism.
Amino acids may have been formed naturally in early earth history. In a process called photochemical dissociation, ultraviolet radiation is capable of separating the atoms in compounds such as water, ammonia, and hydrocarbons such as methane, allowing these atoms to recombine. Some of those atoms would have formed amino acids.
A second form of energy that could trigger recombination of atoms is electrical discharge, occurring naturally as lightning. Lightning and ultraviolet radiation, acting separately or together, could have triggered the formation of amino acids in the atmosphere or in the oceans.
Other Building BlocksAnother of life?s basic components is nucleic acids, such as deoxyribonucleic acid (DNA) and ribonucleic acid (RNA), which transmit genetic information from generation to generation. The building blocks of nucleic acids are nucleotides, which also participate, as nucleotide phosphates like adenosine triphosphate (ATP), in biochemical energy transactions.
Even with the first three components present on the early earth, living organisms could not develop unless those components were in close proximity to one another so they could interact.
Some sort of structure was needed to contain all the components. Cell membranes would have provided just such a structure and so must be considered an essential fourth component of the first living organisms.
Wöhler?s ExperimentBefore 1828, many scientists believed that all organic molecules were products of living organisms. However, in 1828 the German chemist Friedrich Wöhler produced crystals of urea quite by accident after heating an inorganic compound called ammonium cyanate.
Urea, a component of urine, is an organic compound. Over the next several decades, other chemists were able to duplicate Wöhler?s experiment and, by using other simple inorganic compounds, succeeded in producing several other simple organic substances.
This led many scientists to believe that life on Earth could have developed from inorganic materials. Between 1828 and the 1960?s, however, scientists were able to produce only simple organic molecules from inorganic substances. It was only during the 1960?s that scientists couldcreate and detect complex protein molecules.
Miller-Urey Experiments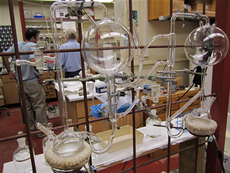 |
Miller-Urey Experiments |
It was not until 1953 that scientists produced amino acids and similar molecules using simulated conditions like those assumed to have been present in the early earth?s atmosphere.
Stanley Miller, a graduate student working for Dr. Harold Urey, created an atmosphere of methane, ammonia, hydrogen, and water vapor in a bottle, thinking that this mixture would have been similar to the atmosphere of the very early earth. As the mixture was circulated through the apparatus, sparks of electricity, to simulate lightning, were discharged into the mixture.
At the end of eight days, the condensed water in the apparatus had become cloudy and deep red. Analysis of the material showed that it contained a number of amino acids along with a few other, more complicated organic compounds.
Other scientists carried out similar experiments, with much the same results, leading many scientists to believe that organic compounds could be produced from a mixture of gases, including a mix of carbon dioxide, nitrogen, and water vapor, also found in Earth?s early atmosphere. What was necessary for the success of these experiments was the lack of free oxygen.
The experimenters felt it almost inevitable that amino acids would have developed in the earth?s pre-life environment. Amino acids are relatively stable and probably became abundant in the early oceans, over time, joining together into more complex molecules.
Amino Acids to ProteinoidsIn order to form proteins, amino acid molecules must lose some water from their molecular structures, which happens when amino acids are heated to temperatures of 140 degrees Celsius. Volcanic activity would have been capable of providing such temperatures.
A biochemist named S. W. Fox and his coworkers were able to produce proteinlike chains from a mixture of eighteen common amino acids. Fox termed these structures proteinoids and thought that billions of years ago proteinoids were the transitional structures leading to true proteins.
Fox actually found proteinoids similar to those he created in the laboratory in lava and cinders spewed out by Hawaiian volcanoes. Amino acids formed in the vapors emitted by the volcanoes and were combined into proteinoids by the heat of escaping gases.
Proteinoids to Microspheres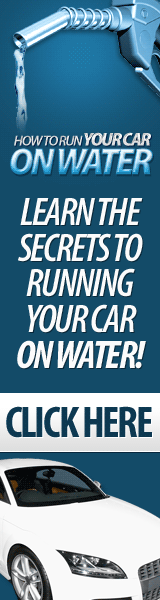 |
running your car on water |
When solutions of proteinoids in hot water are cooled, they form tiny spheres with many characteristics of living cells. These microspheres have a filmlike outerwall, somewhat like a cell membrane; are capable of osmotic swelling and shrinking; exhibit budding, as do yeast cells; and can be observed to divide into ?daughter? microspheres.
Sometimes these microspheres join together to formlines, or filaments, as some bacteria do, and it is possible to observe movement of internal particles within microspheres, similar to cytoplasmic streaming in living cells.
Nucleic Acids in the LaboratoryComplete long-chain nucleic acids have not yet been experimentally produced under pre-life conditions. However, short stretches of nucleic acid components were produced in 1976 by Har Gobind Khorana and his associates at the Massachusetts Institute of Technology.
In yet another experiment, parts of a nucleic acid called peptide nucleic acid, or PNA, were produced by discharging electricity through a blend of methane, ammonia, nitrogen, and water.
PNA is more stable than RNA and may have existed during the earth?s very early days. However, the big questions remain: How did replication of these nucleic acids begin? How did the first organisms manage to pass along their genetic information to the next generation?
Life Begins in the SeaThe earliest organisms apparently originated in the sea, which contains many of the organic compounds, minerals, and other nutrients needed by living organisms. This reflects the viewpoint of many scientists, including Charles Darwin, who said in 1871 that life started in a ?warm little pond,? and Aleksandr I.
Oparin, who proposed in the 1930?s that many chemical substances would have washed out of the atmosphere and been carried into the oceans by rain, creating a ?primordial soup? of nutrients for the first organisms. These organic molecules, kept in constant motion by the ocean currents,may have bumped together, recombining into larger molecules and increasing in complexity.
Exactly how, or exactly when, the transition from not-quite-living to living took place is not known, but scientists assume that it could not happen under present-day conditions. Oxygen and microbial predators would destroy similar structures today.
HeterotrophsThe first living organisms were microscopic in size and unicellular. These earliest forms of life were not able to make their own food but instead assimilated small pieces of organic molecules present in the surrounding waters. They no doubt ate one another as well.
Organisms with this type of nutritional mechanism are called heterotrophs. The food of these ancient organisms was digested externally by excreted enzymes before being ingested and metabolized by fermentation, a process that does not require free oxygen.
Autotrophs and PhotoautotrophsWhen nearby food resources became exhausted, the resulting food shortages might have caused selective pressures for evolutionary change. Some organisms evolved the ability to synthesize their food from simple inorganic substances.
These became organisms called autotrophs. The autotrophs began to evolve inmany different directions, using different substances, such as carbon dioxide, ammonia, and hydrogen sulfide, as food.
Another group evolved into the photoautotrophs, capable of carrying out photosynthesis, which uses solar energy to incorporate carbon dioxide into organic molecules and releases oxygen as a byproduct.
Carbon combined with other elements to promote cellular growth, and the oxygen escaped into the atmosphere, which prepared the environment for the next important step in the evolution of primitive organisms.
As the photoautotrophs multiplied, photosynthesis began to gradually change Earth?s original oxygen-poor atmosphere to a more oxygen-rich one.
A rapid buildup of oxygen in the atmosphere was delayed, as iron in rocks exposed at the earth?s surface was oxidized before oxygen could accumulate in the atmosphere. This allowed many microorganisms to evolve oxygen-mediating enzymes that permitted them to cope with the new atmosphere.
Once atmospheric oxygen concentrations reached about 10 percent, solar radiation converted part of the oxygen to ozone, forming a shield against ultraviolet radiation. Life, still primitive and vulnerable, was now protected and could expand into environments that formerly had not been able to harbor life, setting the stage for the appearance of aerobic organisms.
Aerobic MetabolismAerobic organisms use oxygen to convert their food into energy. Aerobic metabolism provides far more energy in relation to food consumed than does the fermentation carried out by anaerobic organisms. Aerobic metabolism provided a surplus of energy, which was an important factor in the evolution of more complex forms of life.
With more energy available, organisms could move about more, colonize new niches, and engage in sexual reproduction, allowing new and innovative genetic recombinations to emerge, and may have increased the rate of evolution, which led to the evolution of complex multicellular organisms called metazoans.
Prokaryotes and EukaryotesThe first organisms were unicellular organisms called prokaryotes, which today are classified in two of the three domains of life as either Archaea or Bacteria, the latter including the cyanobacteria, which are photosynthetic. Both of these domains comprise unicellular life-forms consisting of prokaryotes. Prokaryotic cells lack internal organelles and a membrane-bound nucleus.
Their genetic material resides in the cytoplasm of the cell. Modern prokaryotes do have cell walls and most are able to move about. Prokaryotes are asexual, reproducing by binary fission, which limits the possibilities for variation.
Evolution proceeded from the prokaryotes to organisms with a definite nuclear wall, well-defined chromosomes, and the capacity to reproduce sexually. These more advanced life-forms are termed eukaryotes.
Eukaryotic cells contain organelles such as mitochondria, which metabolize carbohydrates and fatty acids to carbon dioxide and water, releasing energy-rich phosphate compounds in the process. Some eukaryotes have organelles called chloroplasts, the structures in which sunlight is converted into energy in the process of photosynthesis.
The forms of life made of eukaryotic cells are now classified in the third great domain of life, Eukarya,which comprises most of the life-forms familiar as plants and animals?from fungi and plants to human beings?classified in several kingdoms, phyla, classes, genera, andmillions of species.
Biologists believe that the organelles in eukaryotic cells were once independent microorganisms that entered other cells and then established symbiotic relationships with the primary cell. This process is called the endosymbiont theory.
Ancestral AlgaeThe various divisions of algae make up an important group of photosynthetic eukaryotes. One group of algae, the Chlorophyta, or green algae, are the probable ancestors of terrestrial plants.
Common characteristics of green algae and terrestrial plants include the chlorophyll pigments a and b in the chloroplasts of both green algae and land plants, a number of carotenoid pigment derivatives, starch as the carbohydrate food reserve, and cell walls made up of cellulose.
From Sea to LandIf, as scientists believe, life originated in the sea, howdid it get onto the land? Did the ongoing push to find new sources of food drive organisms from the depths of the oceans to coastal areas, where environmental conditionswere harsher?
To cope with the intense wave action along shorelines, multicellular organisms with diversified parts, some designed to hold onto rocks, evolved. To prevent desiccation in coastal environments and to provide support against the pounding waves, these organisms also evolved rigid cell walls, which allowed them to increase in size.
As plants increased in size, they had adapt to do two things: First, they needed to be able to move water and mineral nutrients from the substrate to other parts of the plant not tied directly to the ground.
A second problem involved how to move the products of photosynthesis from the site of manufacture to those parts of the plant where photosynthesis could not take place.
The solution to these problems became more critical as plants began to colonize habitats farther from permanent supplies of water. Roots evolved to anchor plants in the ground and to supply the rest of the plant with water and mineral nutrients from the ground.
Stems evolved to provide support for the leaves, themain organs of photosynthesis; in some cases, the stems themselves evolved the capability of photosynthesis. All of the above ground portions of plants developed a waxy cuticle that slows down water loss and prevents desiccation.
Internally, plants evolved specialized tissues, called the vascular tissues.One type of vascular tissue, the xylem, conducts water and mineral nutrients fromthe soil through the roots and stems to the leaves. The other type of vascular tissue, the phloem, transports the sugars manufactured in the leaves during photosynthesis to other parts of the plant body.
Many plants also evolved chemical attractants and defenses in the form of secondary metabolites: metabolic products, such as alkaloids, glycosides, or saponins, to deter plant-eating animals.
With the appearance of flowering plants (angiosperms), plants began to evolve odors, colors, flowers, and fruits that attracted pollinators and herbivores, ensuring seed dispersal and the survival and propagation of flowering species. It was these specializations that allowed plants to move from the sea and colonize the land between 500 million and 450 million years ago.
-
Anaerobes And Heterotrophs
AnaerobesThe first organisms to evolve on the earth are thought to have been heterotrophs and anerobes. Heterotrophs are organisms that cannot produce their own food but must fill their energy requirements by consuming organic molecules produced by other...
-
Anaerobic Photosynthesis
Anaerobic PhotosynthesisAnaerobic photosynthesis, also known as anoxygenic photosynthesis, is the process by which certain bacteria use light energy to create organic compounds but do not produce oxygen. Anaerobes are those bacteria that cannot use oxygen...
-
Calvin Cycle
Calvin cycleThe Calvin cycle is the principal mechanism that leads to the conversion of carbon dioxide into sugars by plants, algae, photosynthetic bacteria, and certain other bacteria that use chemicals as an energy source instead of light. The Calvin...
-
Carbon Cycle
The carbon cycle is the movement of the element carbon through the earth?s rock and sediment, the aquatic environment, land environments, and the atmosphere. Large amounts of organic carbon can be found in both living organisms and dead organic material....
-
Microbial Nutrition And Metabolism
Microbial Nutrition and MetabolismThe diverse metabolic activities of microorganisms make them a critical component of all the earth?s ecosystems and a source of many useful products for human industry. Microorganisms?bacteria, fungi, algae, and protists?are...
Agriculture