Agriculture
Liquid Transport Systems
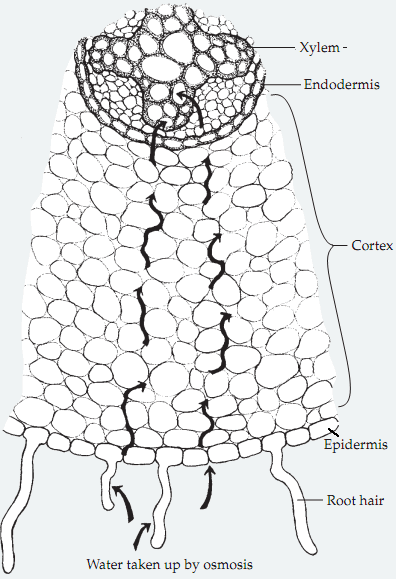 |
Liquid Transport Systems |
Liquid transport systems are structures that facilitate the movement of water, via the xylem, from a plant?s roots to its leaves. Water then evaporates from the leaves through the stomata in the process of transpiration.
Water is themost abundant compound in plant cells. It accounts for 85-95 percent of the weight of most plants. It even makes up 5-10 percent of the weight of ?dry? seeds. More than 95 percent of the water gathered by a plant, however, evaporates back into the atmosphere, often within hours after being absorbed. This evaporation of water from a living plant is called transpiration. Most transpiration is from leaves.
Plants transpire huge amounts of water. On a warm, dry day, an average-size maple tree transpires more than 200 liters per hour, while herbaceous plants transpire their own weight in water several times per day.
A corn plant transpires almost 500 liters of water during its four-month growing season. If humans required an equivalent amount of water, a person would have to drink approximately 40 liters per day.
Plant survival depends on the ability to transport water and dissolved materials. Inside individual cells, diffusion is usually adequate for this movement. Small molecules can diffuse across a 50-micrometer-wide cell in less than one second.
 | Botany for Gardeners Third Edition |
 |  | Botany: An Introduction to Plant Biology |
 |
However, diffusion is inadequate for transport from one part of a multicellular plant to another. Multicellular plants must absorb and transport large amounts of water and dissolved minerals from the soil to their leaves,which may be many meters away from the soil.
Multicellular plants must also have a system for transporting the sugars produced in leaves to distant sites for storage and use. Thus, the multi cellular nature of plants was largely responsible for the evolution of xylem and phloem, the two long-distance transport systems in plants.
Leaves and RootsLeaves are the primary photosynthetic organs of most plants. The rate of gas exchange for photosynthesis depends, among other things, on the amount of available surface area.
The loose internal arrangement of cells in leaves produces a large internal surface area for transpiration an area that may be more than two hundred times greater than the leaf?s external surface area.
The internal surface area of a leaf is connected with the atmosphere via an extensive system of intercellular spaces, pores called stomata, that occupy as much as 70 percent of a leaf?s volume.
Stomata are so numerous that a typical leaf of a squash plant has more than eighty million of them. Leaves also have an efficient system of veins (vascular tissue) for distributing water to their internal evaporative surfaces. One square centimeter of leaf may have as many as six thousand outlets of vascular tissue.
Water lost via transpiration must be replaced by water absorbed from the soil by the plant?s roots. The movement of water from the roots to the leaves is very rapid. Water molecules may move as fast as 75 centimeters per minute, which is roughly equivalent to the speed of the tip of a second hand sweeping around a wall clock.
Water and its dissolved minerals move from roots to leaves in xylem. The two kinds of cells in xylem that carry water are tracheids and vessels. Both of these cell types are hollow and dead at maturity. They have thick cell walls and can therefore withstand the fluctuations in pressure associated with water flow.
Tracheids are usually long (up to 10 millimeters) and thin (10-15micrometers in diameter) and overlap one another. Their walls have numerous thin areas that link adjacent tracheids into long, water conducting chains.
Vessels are shorter and much wider than tracheids. The walls separating adjacent vessel elements are often wholly or partially dissolved. Because of their larger diameter and dissolved walls, water moves faster in vessels than in tracheids.
This increased flow rate in vessels may help explain why angiosperms dominate today?s landscapes: Flowering plants, such as grasses, contain tracheids and vessels, while gymnosperms, such as pines, contain only tracheids. In woody plants, the xylem that transports water and dissolved minerals makes up the wood of the trunk.
Water Flow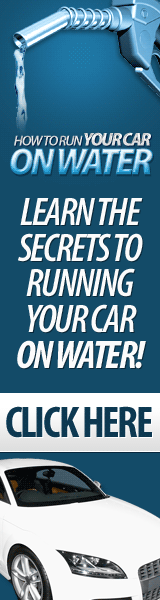 |
running your car on water |
Water movement through plants requires no metabolic energy; rather, water flows passively from one place to another. Although root pressure, caused by the pumping of dissolved minerals into the roots, can push water up to a few meters, in taller plants water and dissolved minerals are pulled up through the xylem. The driving force for this movement is the transpiration of water from the leaves.
The hypothesis that describes the process is known as the transpiration-cohesion hypothesis of water movement. It states that solar-driven transpiration of water dries the walls of mesophyll cells of leaves; the loss of water from the cell wall then causes water from neigh boring cells to enter the leaf cell.
Cells bordering tracheids and vessels replace their water with water from the xylem. The loss of water from xylary elements creates a negative pressure, thereby lifting the water column up the plant. The water column does not break, because water molecules cohere strongly.
The negative pressure created in the xylem by transpiration extends all the way down to the tips of roots, even in the tallest trees. The tension in the root xylem causes water to flow passively from the soil, across the root cortex, and into the xylem of the root. This water is then pulled up the xylem to leaves to replace water lost via transpiration.
Transpiration is affected by atmospheric humidity, wind, air temperature, soil, light intensity, and the concentration of carbon dioxide in the leaf. Transpiration is greatest in plants growing in moist soil on a sunny, dry, warm, and windy day.
In these conditions, transpiration often exceeds the plant?s ability to absorb water. As a result, many plants wilt at midday, even if the soil in which they are growing contains abundant water. Transpiration also moves solutes in plants; for example, most minerals move from roots to shoots in the transpiration stream.
StomataStomata regulate gas exchange between the atmosphere and a plant and are a key adaptation to life on land. Almost all the factors that affect transpiration do so by influencing the opening or closing of stomata. For example, decreasing the internal concentration of carbon dioxide in a leaf causes stomata to open, therefore increasing gas exchange and transpiration.
Stomata occur throughout the plant kingdom. In angiosperms, they occur on all above ground organs, including leaves, stems, petals, stamens, and carpels. Open stomata occupy less than 2 percent of a leaf?s area. In most plants the stomata are confined to the lower surfaces of leaves (away from the sun) to reduce transpiration rates. Stomata on the upper surfaces,where direct sunlight strikes, could cause serious water loss.
A stomatal complex consists of two guard cells and adjacent epidermal cells called subsidiary cells, all of which surround a pore. Guard cells and stomata have several distinguishing features. For example, guard cells of dicotyledons are crescent shaped, while in most grasses they are shaped like dumbbells. Most leaves have 1,000 to 100,000 stomata per square centimeter of leaf area.
Plants in dry, bright environments, such as deserts, often have smaller and more numerous stomata than plants growing in wet, shaded environments. When wide open, stomatal pores are usually 3-12 micrometers wide and 10-40 micrometers long.
Guard cells control the size of a stomatal pore by changing shape?their unusually elastic walls buckle outward when stomata open and sag inward when stomata close. Stomatal opening and closing is controlled by movement of water into and out of the guard cells.
The water movement occurs by osmosis, and the direction of movement is determined by the concentrations of ions under a complex set of cellular controls. Stomatal opening is primarily caused by radial micellation of guard cells by cellulose microfibrils arranged much like the belts in a belted tire.
These microfibrils are inelastic; they restrict radial expansion of guard cells while allowing increases in length. When the guard cells lengthen because of an influx of water, they bow apart and form the stomatal pore. Closing of stomata occurs when water moves out of the guard cells, causing them to become flaccid.
Sieve Tubes and SugarSugars and other organic substances move in sieve tubes of the phloem. Sieve tube members are arranged end-to-end and are associated with files of companion cells. Companion cells and sieve elements function as a single unit.
Sieve tube members are tiny cylinders approximately 40 micrometers in diameter and 1,200 micrometers long. The protoplasts of sieve tube members are connected by sieve like areas called sieve plates, each of which has numerous sieve pores. In woody plants, the phloem makes up the innermost layers of the bark.
Peak rates of solute transport in phloem may exceed 2meters per hour.As a result, as much as 20 liters of sugary sap can be collected per day from severed stems of sugar palms.
A sieve element 0.5 millimeter long empties and fills every two seconds, thereby delivering approximately 5 to 10 grams of sugar per hour per square centimeter of phloem area to sites of sugar storage or use.
Solutes move through the phloem via pressure flow. In 1926 plant physiologist Ernst Munch proposed the pressure-flow hypothesis, which states that a turgor pressure gradient drives the unidirectional mass flow, or bulk flow, of solutes and water through sieve tubes of the phloem.
According to this model, solutes move passively through sieve tubes along a pressure gradient in a fashion analogous to the movement of water through a garden hose.
Sucrose produced at a source is actively loaded into a sieve tube. This loading causes water to enter the sieve tube via osmosis from the xylem. The influx of water into sieve tubes carries sucrose to a sink, which is an area where sucrose is unloaded for use or storage.
Removing sucrose at the sink causes water also to move out of the sieve tube. The influx of water at the source and the efflux of water at the sink create a pressure-driven flow of fluids in the phloem.
Sugars in the cell wall are loaded into sieve tubes by companion cells. These companion cells often have numerous cell-to-cell channels called plasmodesmata. Their cell walls and cell membranes also have elaborate in foldings that provide a large surface area for transporting sugars from the cell wall into the sieve tube.
The loading of sieve tubes requires metabolic energy in the form of adenosine triphosphate (ATP) and is driven indirectly by a proton gradient. Phloem transport is affected by temperature, light, and the nutritional status of the plant.
More than 90 percent of the solutes in sieve tubes are carbohydrates. In most plants, these carbohydrates are transported as sucrose. The concentration of sucrosemay be as high as 30 percent, thereby giving the phloem sap a syrupy consistency. A few plant families also transport other sugars, such as raffinose and stachyose.
These sugars are similar to sucrose and consist of sucrose attached to one or more molecules of D-galactose. Like sucrose, all these sugars are nonreducing sugars. This is important because nonreducing sugars such as sucrose are less reactive and less prone to enzymatic breakdown than are reducing sugars such as glucose and fructose.
Sieve tubes also contain ATP and nitrogen-containing compounds. More than a dozen different amino acids occur in sieve tubes of some plants. Sieve tubes also transport hormones, alkaloids, viruses, and inorganic ions such as potassium ions.
-
Angiosperm Cells And Tissues
Some cell types and tissues which are not found in any other groups of plants occur in angiosperms (flowering plants). Angiosperms are a group of plants with seeds that develop within an ovary and reproductive organs in flowers. They are commonly referred...
-
Cells And Diffusion
Cells and DiffusionPlant cells, like all other living cells, are surrounded by a semipermeable membrane, and any particle moving into or out of the cell must cross this membrane. There are three basic processes by which particles move across plant cell...
-
Gas Exchange In Plants
Gas Exchange in PlantsGas exchange is the process whereby water vapor and oxygen leave and carbon dioxide enters plant leaves. The gaseous balance in plants is quite complex because plant cells carry on both respiration and photosynthesis. All living...
-
Leaf Anatomy
Leaf Anatomy The leaf has evolved as the chief part of the plant for gathering light energy from the sun and conducting photosynthesis to transform that light energy into biochemical energy. Hence, its structure is adapted to that function. Leaves are...
-
Plant Tissues
Plant TissuesPlant tissues are the distinctive structural and functional units of a plant that carry out all its basic life functions, including growth, reproduction, support, metabolism, circulation, and protection from the environment. The body plan...
Agriculture